SUNDAY, 5 MARCH 2017
Gianamar Giovannetti-Singh explores the holographic universeModern fundamental physics consists of two major pillars; general relativity, describing the interactions between matter and spacetime at the largest scales imaginable, and quantum mechanics, the physics governing the behaviour of subatomic particles. Despite each respective theory being tested to an extraordinary degree of accuracy, they are fundamentally incompatible with each other – general relativity predicts continuous spacetime as the fabric of the universe whereas quantum physics deals exclusively with discrete, quantised units of matter and energy.
Theoretical physicists generally consider quantum gravity, a successful amalgamation of the two theories, to be the holy grail of modern physics, as it would unify these two immiscible faces of the universe into a so-called theory of everything, capable of predicting the behaviour of every structure in the universe within a single mathematical framework. Notable attempts at formulating a quantum theory of gravity include string theory, in which all the point-particles of the standard model of particle physics are replaced with one-dimensional strings, and loop quantum gravity, which breaks up spacetime, the fabric of our universe, into discrete chunks with a size of approximately 10-35 metres – if a hydrogen atom were the size of the sun, this would be the size of a single proton. Unfortunately, all of these attempts have proved to be far less successful than expected, offering no real falsifiable hypotheses. However, an alternative and rather radical approach to quantum gravity has recently been gaining increasing recognition in the physics community following a sudden inflow of supportive evidence from computer simulations; this approach is known as the holographic principle. This principle argues that rather than living in a three-dimensional universe which we perceive every day, the cosmos is actually a projection of three-dimensional information onto a two-dimensional surface – not dissimilar from the way in which a hologram encodes information about three-dimensions on a 2D surface. The holographic principle was suggested by Gerard ‘t Hooft as a consequence of Stephen Hawking and Jacob Bekenstein’s ground breaking work on black hole thermodynamics in 1974, which demonstrated that the entropy (a measure of disorder) of a black hole varies not with its volume as one might naïvely expect, but rather with its surface area. As entropy is intrinsically linked to information, ‘t Hooft proposed that the information of a 3D object is simply encoded on a 2D surface. The great American physicist and science communicator Leonard Susskind developed ‘t Hooft’s idea and formalised it in the mathematical framework of string theory, allowing it to be fully integrated within another physical model.
In 1997 Juan Maldacena used Susskind’s mathematical framework to derive a tremendously profound result – if the “laws” of physics which followed from the holographic principle (namely that information about an N-dimensional system can be encoded on an (N-1)-dimensional surface without losing any knowledge of the system) are applied to a physical theory which describes how gravity would behave in a 5D universe, the results agree completely with a quantum-based theory (Yang-Mills theory) in four dimensions. This was an initial clue that the holographic principle holds great power in its ability to unify seemingly completely detached areas of physics, and in its combination of theories derived from general relativity and quantum mechanics, the principle secured its status as a candidate for a theory of quantum gravity.
"...the holographic principle holds great power in its ability to unify seemingly completely detached areas of physics"
A major piece of evidence in favour of the holographic principle was obtained in late 2013 by a team led by the Japanese theoretical physicist Yoshifume Hyakutake et al., who ran two very large simulations on a supercomputer calculating the internal energy of a black hole using two completely different physical models; one was a ten-dimensional universe based on general relativity, the other was a one-dimensional universe without gravity but rich in large-scale quantum effects. Somewhat astoundingly, Hyakutake et al. found that the two values agreed exactly, which lends credence to the holographic principle; i.e. that a gravitational model of spacetime corresponds directly with a lower-dimensional quantum-dominated universe. This simulation has significantly improved the standing of the holographic principle as a candidate for quantum gravity as it has demonstrated a universal correspondence between a theory derived from general relativity and a lower-dimensional quantum theory, and so far provides the only quantitative link between the two regimes. Whilst models such as string theory and loop quantum gravity attempt to quantitatively describe both gravitational and quantum behaviour, neither provide any numerical values which can be falsified, whereas Hyakutake et al.’s work did just that, and thus allow the holographic principle to present (somewhat more falsifiable) hypotheses regarding the internal energy of a black hole.
Whilst the nature of a theory of quantum gravity remains elusive to physicists at present, the holographic principle may just be the dark horse in the race for grand unified theory of nature.
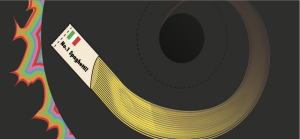