MONDAY, 30 MARCH 2020
From the latest Star Wars film to the 50th Moon Landing anniversary celebrations, you don’t need to look far to find people excited by the idea of space travel. Interest in visiting planets other than our own is nothing new. In fact, the earliest known example of a novel featuring space travel was called ‘A True Story’ and was written in the second century AD by Lucian of Samosata. However, the idea of spaceflight as a scientific endeavour took longer to develop and it is not until 1865 in Jules Verne’s ‘From Earth to the Moon,’ that a realistic vision of spaceflight materialised in fiction.Jules Verne’s novel is the story of how Impey Barbicane uses his position as president of the Baltimore Gun Club to build the Columbiad, a cannon capable of firing people to the moon. Aside from being an early example of science fiction, the book is noteworthy due to the rigorous way in which Verne approached his subject matter. This enabled him to make a number of accurate predictions, including which state the first manned voyage would take off from! In fact, Verne’s novel was so prescient that NASA decided to honour it by naming the Apollo 11 command module ‘Columbia’, after the Columbiad.
NASA was less eager to recognise the other precursor to its Apollo missions. The technology used to send the first men to the moon was a direct descendant of the V2 rockets used by Nazi Germany in World War II. After the war, the designers of the V2 rocket, Hermann Oberth and Werhner von Braun both moved to America where their work led to the development of the Saturn V rocket and so, indirectly, to the moon landings.
Oberth, along with Konstantin Tsiolkovsky and Robert H. Goddard are considered to be the fathers of modern rocketry. Tsiolkovsky formulated what is now known as the ‘ideal rocket equation’ which states
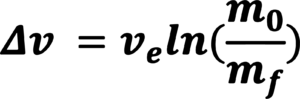
In words this means that the amount by which a rocket can change its velocity (Δv), is limited by two factors. One is the exhaust velocity (ve ) - the velocity at which the exhaust comes out the back of the rocket. The other is the ratio of the rocket’s initial mass (m0 ) to its final mass (mf ). The equation is a consequence of the method by which a rocket propels itself. Rockets work by ejecting a portion of their mass, normally in the form of a fast-moving gas. When the gases are ejected, the rocket exerts a downward force on them. By Newton’s Third Law, the gases must exert an equal and opposite force upwards on the rocket and this is the force that accelerates the rocket.
Tsiolkovsky’s equation explains the need for multistage rockets. For a fixed exhaust velocity, the greater the ratio of the rocket’s initial mass to its final mass, the larger the Δv it can achieve. Rockets need to achieve a Δv large enough that they can escape the Earth’s gravitational pull. If one tried to achieve this Δv with a single stage rocket powered by conventional propellants, Tsiolkovsky ‘s equation shows that a prohibitively large amount of the rocket’s initial mass would have to be taken up by fuel, leaving little solid mass left over for the payload. In a multistage rocket, each stage contains its own engine and supply of fuel and once the stage has finished burning it detaches from the rest of the rocket. This has two consequences: First, the later stages start from a higher initial velocity and so have to achieve a smaller Δv to reach the final desired velocity. Second, later stages have less mass to accelerate as the useless mass of the previous stage’s engine has been offloaded. Until recently, the fate of these early stages had been to fall back into the ocean or an uninhabited region of Earth. However, due to efforts to make spaceflight more commercially viable, these early stages are now being collected and reused.
Robert H. Goddard is remembered as the first man to ever launch a liquid-propellant rocket. In general, one can fuel a rocket using either a liquid or a solid propellant. Solid-propellant rockets have a ‘fuel cake’ where the propellant and oxidiser have already been mixed. This solid fuel is then ignited to produce the gases that propel the rocket forward. The earliest forms of rockets, such as cannons, firework displays and Jules Verne’s hypothetical Columbiad, worked in this manner. Liquid-propellant rockets contain both fuel and an oxidiser in a liquid state. These are then brought together in a combustion chamber where the ignition takes place.
Goddard is remembered because liquid-propellant rockets have several advantages over their predecessors, especially for applications to manned spaceflight. As the flow of fuel and oxidiser to the engine can be tightly regulated, it is possible to control exactly how much thrust is produced at any given time, which is vital in performing delicate space manoeuvres. Additionally, liquid-propellants tend to have a higher specific impulse than their solid counterparts. Specific impulse is a measure of how much thrust a given amount of propellant delivers to a rocket and is equal to the exhaust velocity. As specified by Tsiolkovsky’s equation, a higher exhaust velocity results in a greater Δv, so rockets with higher specific impulses can afford to carry greater payloads. Lastly, liquid-propellant rockets have a much shorter turnaround time between uses than solid propellant rockets, making them more attractive to the likes of Elon Musk and SpaceX, who aim to develop space tourism.
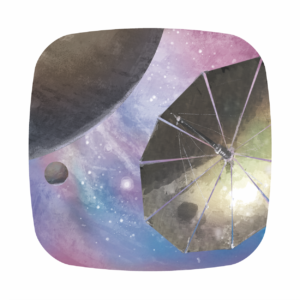
Chemically powered rockets, whether based on a solid or liquid-propellant, currently provide the best way of escaping the Earth’s atmosphere as they have a high thrust-to-weight ratio. This is a measure of the thrust that a particular engine can provide divided by the total weight of that engine. In order to escape the Earth’s gravitational pull, a rocket must have a thrust-to-weight ratio of greater than one. However, there are inherent limits to how far away from Earth we can travel using these means of propulsion since chemical reactions have a relatively low energy density To really push out into space and explore the final frontier we need to move to new forms of propulsion.
Ion thrusters have been proposed as a viable alternative to chemical propulsion systems. These generate thrust by ionising the gas in the rocket’s engine and then accelerating it to high velocities using electric fields. The acceleration that can be achieved depends on the ion’s charge to mass ratio, which is large, and so relatively small electric fields can produce large accelerations. As the ions can be accelerated to such high velocities, the exhaust velocity is much greater than for chemically powered rockets and so a much larger Δv can be achieved. The downside to ion thrusters is that they have a low thrust-to-weight ratio. This means that, although the Δv of an ion thruster is large, in reality it will take a very long time to achieve that Δv. Solar sailing has also been proposed as an alternative mode of space travel. Like ion thrusters, this method is unable to generate large amounts of thrust but has the advantage that, once in space, these rockets would have to carry no fuel. This is because they are accelerated by the pressure exerted on their sails by the sun’s radiation.
Both of these methods are similar as they are unable to escape the Earth’s gravity well unaided, making them best suited to voyages between worlds. Due to their low thrust, it would take years for craft powered by these systems to build up any appreciable velocity. However, given that our nearest neighbouring star system is four light years away, time is not something that would be lacking in an interstellar voyage. To truly travel among the stars may take years or even lifetimes. However, in my opinion, the human desire to see and explore what is out there will overcome even this obstacle.
Lucy Hart is a third year Physical Natural Sciences student at Peterhouse. Artwork by Charlotte Airey.