FRIDAY, 7 JULY 2017
Humans have always been captivated by the beautiful bright speckles of light scattered across the night sky and the prospect of exploring these unknown worlds beyond ours. erefore, it is not surprising that the sciences pertaining to outer space (astronomy and its derivatives astrophysics, astrochemistry, astrogeology, and astrobiology) have successfully appealed to a wide audience. This is likely to become even further intensi ed given the recent surge of interest in the exploration of Mars.To date, samples from Mars have never returned to Earth and no human has ever touched the surface of the Red Planet. But this is likely to change soon. NASA’s Mars 2020 Rover aims to collect samples of soil and rock and cache them on Mars’s surface, to be returned to Earth by a future mission. Additionally, according to the Journey to Mars report, NASA aims to send humans to Mars in the 2030s.
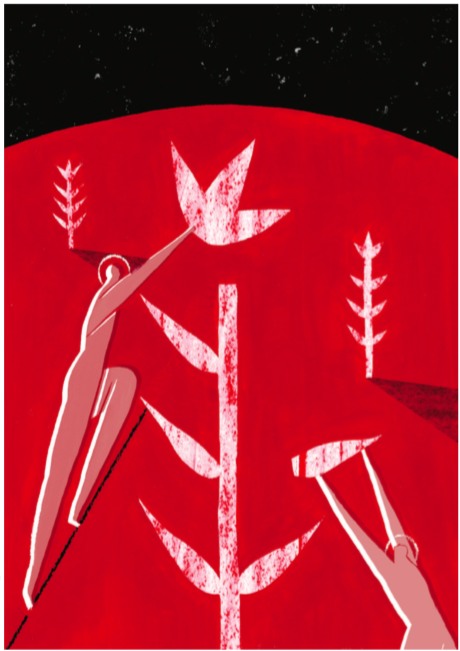
While pondering the most significant steps in the evolution of life, Elon Musk said that “there was the advent of single-celled life, the differentiation to plants and animals, there was life going from oceans to land, there was mammals, consciousness. And I would argue, also on that scale should fit life becoming multiplanetary.” Indeed, making humans an interplanetary species would allow us to avoid a potential mass-extinction event localised to one planet, and could also help us understand more about our solar system and universe.
However, there are many questions we need to answer before we become interplanetary and set up a Mars colony. First, what will the colonists do on Mars? What will the economy look like? It is clear that the first colonists will rely heavily on resources from Earth to sustain themselves, and the possibility of ever creating a self-reliant settlement in such an inhospitable world is itself debatable. Surface temperatures can range from -17°C to -143°C, and the thin atmosphere means radiation poses a real problem. Second, how should we choose the rst settlers? Whose laws will they follow? Who will own Mars? Article II of the UN Outer Space Treaty prohibits countries from claiming territories in outer space including the Moon and other celestial bodies. But this may exclude private individuals and companies. erefore, one view is that we should, similar to the rst settlers of the New World, simply let the rst Martian settlers start their own civilisation anew. Let them write their own Martian Constitution.
This creates its own set of problems. It is highly unlikely that single private individuals or companies would be able to fund a Martian colony. Price estimates for a human Mars mission have ranged from $6bn for Mars One’s one-way trip, to Musk’s $10bn (for rocketry alone), to $100bn according to a 2014 review by an expert NASA panel. The $100bn is probably the best estimate. Such a price tag will mean that national governments would have to become involved and national interests would inevitably come into play.
The first people to touch Martian soil will go down in history, but it is important that we think through whether we even want to ‘touch Mars’. In fact, a community of scientists has formed the International Committee Against Mars Sample Return, and even Nobel laureate Carl Woese argued that “when the entire biosphere hangs in the balance, it is adventuristic to the extreme to bring Martian life here. Sure, there is a chance it would do no harm; but that is not the point. Unless you can rule out the chance that it might do harm, you should not embark on such a course.”
He refers to the issue of back contamination, meaning that a sample return could bring back a Martian life form or pathogen that we have not been naturally selected to have resistance against. It is why the Apollo 11 astronauts were quarantined for three weeks after they returned from the Moon.
But what about the reverse process, or forward contamination? This not only has similar implications with Earth life killing potential Martian life, but also creates the need to distinguish between the two. NASA has a number of dedicated people working in the Office of Planetary Protection to reduce the possibility of such forward and back contamination by employing both microbial reduction and microbial monitoring methods.
Mars clearly does not contain any macroscopic life; however, we still cannot rule out the existence of microbial life. Indeed, in 1996 the Antarctic Search for Meteorites (ANSMET) project famously found the Martian meteorite ALH84001, and received a lot of attention when David McKay suggested he had evidence of microscopic fossils based on scanning electron microscopy (SEM) of parts of the meteorite. President Bill Clinton even said in a speech: “Today, rock 84001 speaks to us across all those billions of years and millions of miles, it speaks to the possibility of life. If this discovery is confirmed it will surely be one of the most stunning insights into our universe that science has ever uncovered.” However, it was later shown that similar morphological signatures could be recreated without biological inputs. It would also be reckless to suggest that morphology alone is a sufficient tool to detect primitive life.

In this case, Martian and Earth life would share the same origin, and we could simply perform genomic comparisons to see how the different selective pressures exerted by the different changing environments, separated by vast amounts of time, have resulted in diverged genomes.
Either way, if Martian microbial life exists, an important question we need to ask ourselves is how we should act towards ‘them’. It touches on what we consider to be our purpose in this universe. One view would be that we have no ethical obligation towards Martian microbes at all and are free to terraform Mars however we deem desirable; alternatively, on the other side of the spectrum, we have no right to set foot on Mars (or any other celestial body) and alter it at all. But what if we do find life on Mars, a curious vestige from an era when Mars was better suited for harbouring life? Should we then change Mars to help these organisms flourish?
At the moment, these questions may seem rather futuristic, but with NASA forecast to send the first humans to Mars in the 2030s and with samples likely to return even earlier, these are questions we need to contend with now. Ultimately, the universe would not care if human civilisation was destroyed tomorrow. The laws of physics would not change; everything would be the same. The universe would continue expanding, galaxies would continue moving beyond the observable universe, and distant planetary worlds would continue orbiting their stars. But whatever our purpose, sustaining and conserving Life could be up to us, and to do this effectively space exploration must be interdisciplinary.
The most significant missions will draw on expertise from and collaborations with people from backgrounds in engineering, science, philosophy, law, and politics. What good does life do the universe? Perhaps none. But we might be its only chance of finding out.
Note: Stereochemistry involves the study of stereoisomers, which are isomeric molecules that differ in their three-dimensional arrangements of atoms in space. L– and D–amino acids represent a certain type of stereoisomerism, known as optical isomerism.This means they are non-superimposable mirror images of each other, also known as enantiomers.With a few exceptions, terrestrial life is known to only use L-amino acids probably due to an initial modest imbalance in the abundance of amino acid enantiomers when life originated, leading to a self-reinforcing evolutionary process to select one over the other. It has been shown that D-amino acids can form functional proteins so the decision between D and L is arbitrary.
Banner image credit: Kevin Gill